Research Interests
Materials

Dielectric elastomers (DEs) represent a class of electro-mechanical transducers consisting of thin elastomer membranes (e.g., silicone, acrylics, natural rubber) coated with flexible electrodes (e.g., carbon-silicone mix, thin metal films) on both sides. When a high voltage is applied to the electrodes of a DE, the stored electric charges are subject to electrostsatic forces which cause a thinning of the membrane and, in turn, an area expansion. In this way, a controllable deformation can be obtained. DEs are characterized by large deformations (higher than 100%), high energy efficiency and density, high flexibility, fast response, lightweight, self-sensing capabilities, and low cost. This unique combination of features permits to develop DE based actuators, sensors, and generators capable of performance not achievable with conventional transducers. Eventually, actuation and sensing can be performed simultaneously by exploiting the so-called self-sensing mode, which allows to implement closed loop control architectures without introducing additional position or force sensors.
Our main research interests in this area are:
- DE material characterization
- DE material modeling
- DE systems development
- DE system modeling, control, and optimization
- DE driving electronics
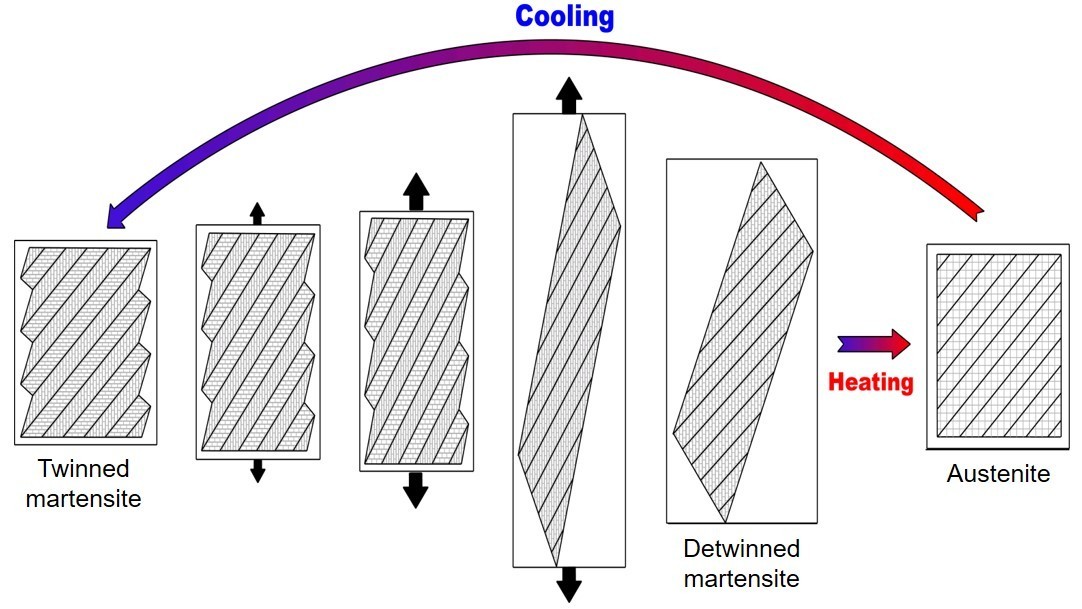
Thermal shape memory alloy (SMA) wires, such as NiTi ones, represent a specific class of smart materials which can be effectively used to design innovative mechatronic actuators. If an electric current is sent through the wire, the resulting Joule heating triggers a phase transformation in the SMA crystal lattice, which results in a change in shape up to 4–8%. If a pre–loading force is applied to the wire, e.g., by means of a pre–tensioned spring, the SMA restores its original shape when the current is removed. Such a principle can be effectively exploited to achieve muscle–like actuation modes. Compared to competing technologies, SMAs are characterized by a high energy density (up to 10 J/cm3), which permits to design compact and lightweight actuator devices. In addition, it is possible to relate the wire resistance to its deformation, enabling the use of SMAs as a self–sensing actuators.
Our main research interests in this area are:
- SMA material characterization
- SMA material modeling
- SMA systems development
- SMA system modeling, control, and optimization
- SMA driving electronics
Methods
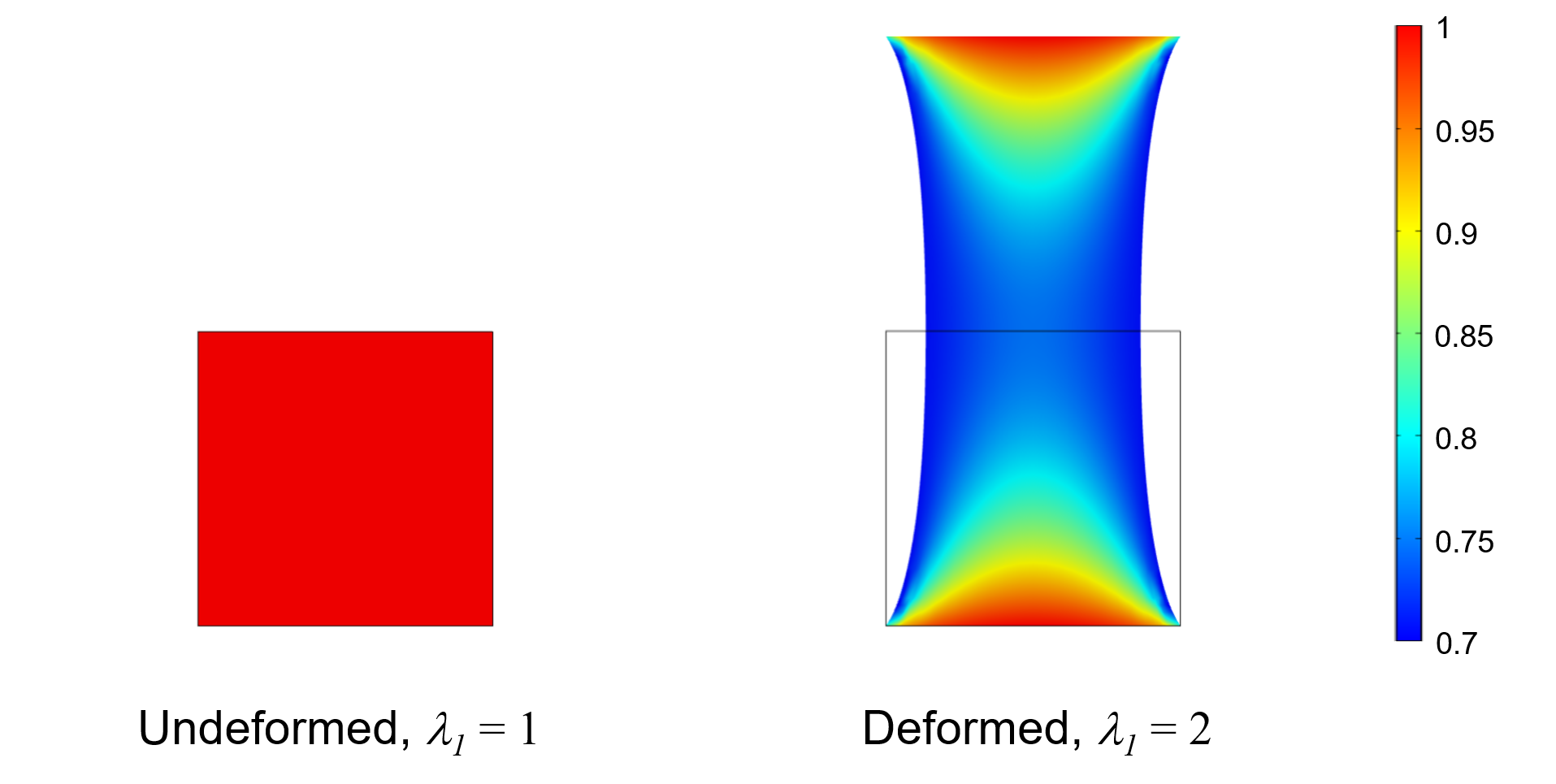
Mathematical models is the starting point for proper understanding, optimization, and control of high-performance smart material systems abnd applications. At APS, the development of mathematical models and simulation tools represents a fundamental part of our research activities. We develop physics-based models based on different techniques and frameworks, which can be exploited for different applications and needs, i.e., optimization, simulation. control. Despite most of our modeling investigations are conducted on smart material transducers and systems, we are also interested in mechatronic systems in general.
Our main research interests in this area are:
- Smart materials modeling
- Smart material actuators, sensors, and generator systems modeling
- Smart material lifetime and damage modeling
- Hysteresis modeling
- Energy-based modeling of multi-physics systems
- Port-hamiltonian modeling
- Hybrid dynamical modeling
- Finite element modeling
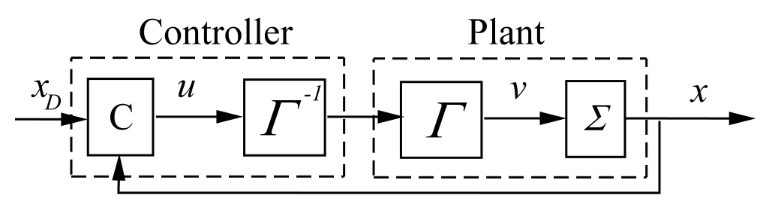
To ensure fast, accurate, and efficient operations in smart material systems, the development of control algorithms is of fundamental importance. At APS, we focus on developing and implementing control algorithms for different types of smart mart material systems and mechatronic actuators in general. Our research on control covers both theoretical and practical aspects, which are relevant for the control of motion, force, and interaction in physicsal systems.
Our main research interests in this area are:
- Control methods for smart material systems
- Hysteresis compensation and control
- Motion control
- Force/interaction control
- Robust control
- Optimal control
- Energy-based control
- Controller implementation
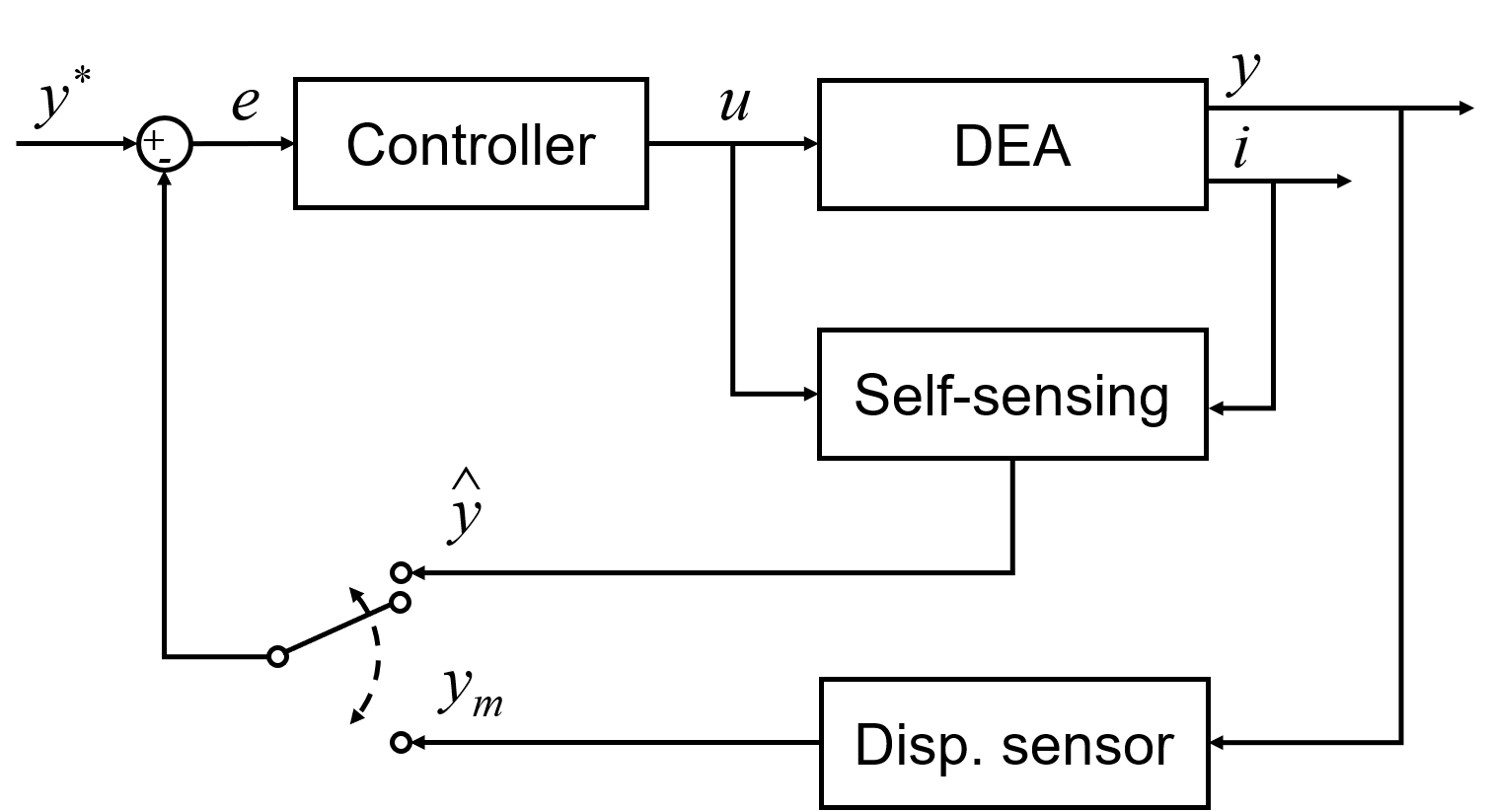
One of the main advantages of smart materials is the so-called self-sensing feature, i.e., the possibility of using the transducer as an actuator and as a sensor at the same time. This operation mode allows to reduce the size and cost of a smart material system, without removing all the benefits of feedback control. At APS, we focus on developing and implementing self-sensing architectures for different types of smart material transducers. By allowing the possibility tio estimate the material geometry and/or force during actuation, we enable the development of truly multifunctional systems.
Our main research interests in this area are:
- Capacitive self-sensing methods for DE
- Reistive self-sensing methods for SMA
- Self-sensing methods for hysteretic systems
- Self-sensing for multi degree-of-freedom smart actuators
- Simultaneous force and displacement self-sensing
- Sensorless control
- Online self-monitoring
- Self-sensing hardware development
- Self-sensing architectures implementation

Experimental validation of the developed control and self-sensing algorithms represents a fundamental aspect of our research. Experimental validation of intelligent algorithms is addressed at APS at two levels. On the one hand, advanced simulink-based FPGA architectures allow rapid prototyping of complex real-time algorithms. On the other hand, the development of integrated smart actuators and applications requires the algorithms to be implemented in compact microcontroller architectures.
Our main research interests in this area are:
- Experimental validation of control & self-sensing algorithms
- Algorithms integration in high-performance FPGA systems for rapid prototyping
- Algorithms integration in real-time microcontroller architectures
Applications
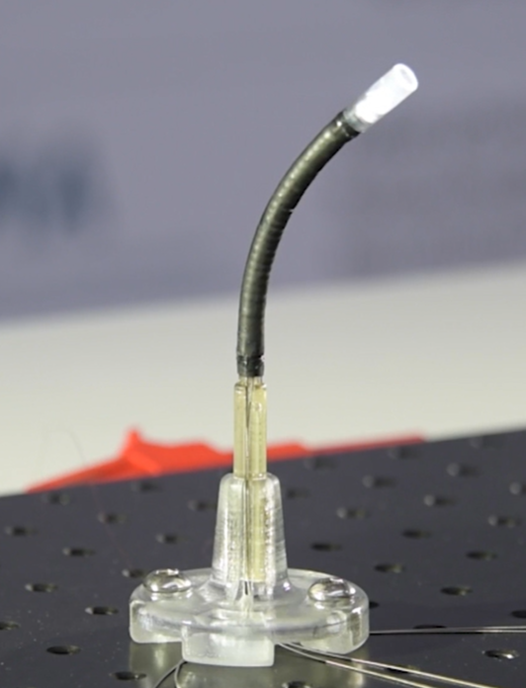
Konventionelle Roboter basieren häufig auf starren Strukturen und Aktoren, um Manipulationsaufgaben auszuführen. Obwohl sie in industriellen Umgebungen weit verbreitet sind, erscheinen starre Roboter aufgrund von mangelnder Flexibilität, hohem Gewicht und hoher Sicherheitsanforderungen moderner Anwendungen oft ungeeignet. Ein relevantes Beispiel ist der Bereich der Mensch-Roboter-Kooperation, in dem starre Strukturen eine Gefahr für einen menschlichen Benutzer darstellen können. Diese neuen Anforderungen haben das Interesse an dem Gebiet der Soft-Robotik geweckt. Dieser Begriff bezieht sich auf Roboter, bei denen inhärent weiche Materialien für die Konstruktion der Struktur sowie der Aktoren-/Sensorkomponenten verwendet werden. Die verbesserten Nachgiebigkeits- und Sicherheitseigenschaften von Soft-Robotern ermöglichen die Entwicklung neuartiger Anwendungen und Paradigmen der Mensch-Roboter-Interaktion. Gleichzeitig ermöglicht die Verfügbarkeit weicher und sehnenartiger Aktoren die Realisierung von Kontinuum-Roboterstrukturen, deren große Anzahl von Freiheitsgraden es erlaubt, komplexe Bewegungsmuster zu erreichen, die mit konventionellen starren Robotern nur schwer oder gar nicht realisierbar sind. Aufgrund ihrer einzigartigen Mischung aus Nachgiebigkeit, großer Verformungen und geringem Gewicht stellen intelligente Materialien wie DE und FGL eine geeignete Technologie dar, um Aktorik und Sensorik in Kontinuum/Soft-Robotern zu realisieren.
Unsere Hauptforschungsinteressen in diesem Bereich sind:
- Weiche Roboter auf Basis von DE-Wandlern
- Kontinuumsroboter auf Basis von FGL-Wandlern
- Neuartige Konzepte für Kontinuum/Weichroboter auf der Basis von intelligenten Materialien
- Entwicklung von Kontinuum/Softroboter-Systemen
- Herstellung von Kontinuum/Softroboter-Systemen
- Charakterisierung von Kontinuum/Softroboter-Systemen
- Modellierung von Kontinuum/Softroboter-Systemen
- Bewegungs- und Interaktionssteuerungsmethoden für Kontinuum/Soft-Roboter
- Self-Sensing-Methoden für Kontinuum/Soft-Roboter
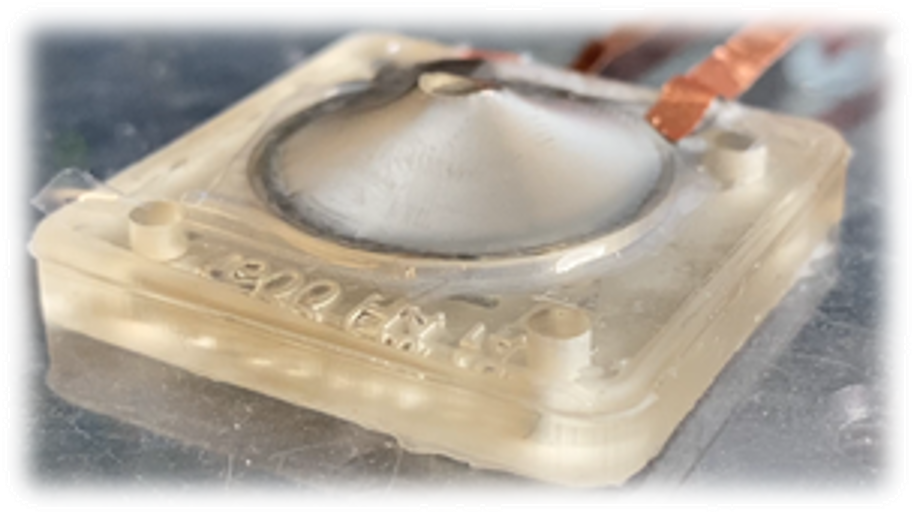
Bis heute basieren die meisten DE-Anwendungen im kleinen Maßstab auf Systemen mit einem Freiheitsgrad, bei denen nur ein aktives Element in einer Stand-alone-Konfiguration verwendet wird. Im Gegensatz dazu kann ein kooperatives System erreicht werden, wenn viele DE-Aktuatoren in einer Array-ähnlichen Konfiguration integriert und in einer synergetischen Weise betrieben werden. Durch die Kombination der intrinsischen Nachgiebigkeit, Energieeffizienz und Self-sensing Eigenschaften von DE-Aktoren mit kooperativen Steuerungsparadigmen kann eine neue Generation von verteilten Mikroaktoren entwickelt werden, die potenzielle Anwendungen in Bereichen wie Soft-Robotik, verteilte Akustik, Wearables und Wellengeneratoren haben, um nur einige zu nennen. Daher (oder: Diesbezüglich) arbeiten wir am APS einerseits an der Entwicklung neuartiger Konzepte für weiche Aktoren und Arrays, und untersuchen andererseits neuartige Modelle und Regelungsalgorithmen, die einen kooperativen Betrieb sicherstellen.
Unsere Hauptforschungsinteressen in diesem Bereich sind:
- Entwurf und Herstellung von vollpolymerbasierten weichen Aktoren
- Entwurf und Modellierung von nachgiebigen bi-stabilen Mechanismen
- Designkonzepte für hochleistungsfähige verteilte Arrays von weichen DE-Aktoren
- Herstellung und Charakterisierung von verteilten Arrays aus weichen DE-Aktoren
- Physik-basierte Modellierung von verteilten Arrays von Soft-DE-Aktoren
- Kooperative Steuerung und Selbsterkennungsalgorithmen für verteilte Arrays von Soft-DE-Aktoren
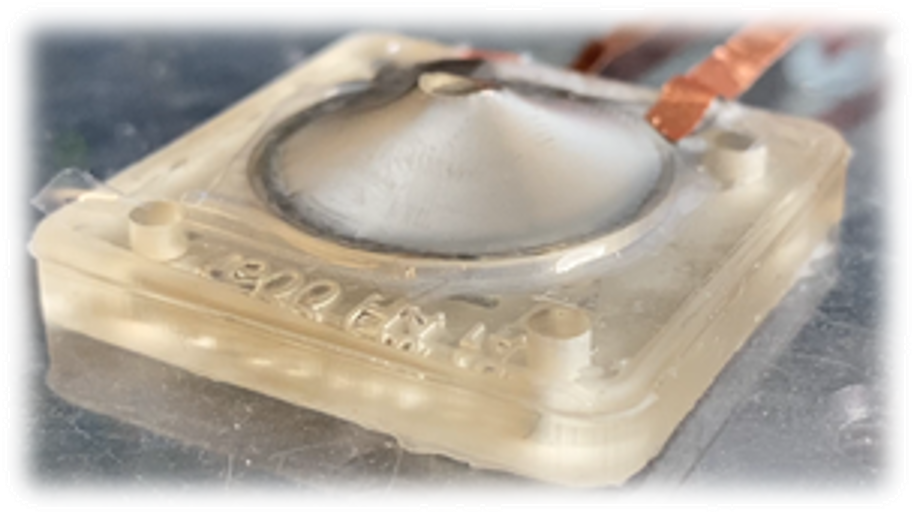
Up to date, most of small-scale DE applications rely on single degree-of-freedom systems, in which only one active element is used in a stand-alone configuration. In contrast, if many DE actuators are integrated in an array-like configuration and operated in a synergistic fashion, a cooperative system can be obtained. By combining the intrinsic compliance, energy efficiency, and self-sensing features of DE transducers with cooperative control paradigms, a new generation of distributed micro-actuators can be developed, with potential applications in fields such as soft robotics, distributed acoustics, wearables, and wave generators, to mention a few. In this perspective, at APS we work on the one hand to developing novel concepts for soft actuators and arrays, and investigate on the other hand novel models and control algorithms which ensure cooperative operation.
Our main research interests in this area are:
- Full polymer-based soft actuators design and fabrication
- Design and modeling of compliant bi-stable mechanisms
- Design concepts for high-performance distributed arrays of soft DE actuators
- Manufacturing and characterization of distributed arrays of soft DE actuators
- Physics-based modeling of distributed arrays of soft DE actuators
- Cooperative control and self-sensing algorithms for distributed arrays of soft DE actuators